Phase Change Materials….what am I missing?

First things first, GBA is a spectacular resource. Thank you to everyone that contributes to this site! Something has been gnawing at me over the past few months and I can’t seem to find clarity, so I’ve finally taken the plunge and gotten a GBA account!
We’re talking Phase Change Materials. PCMs. Everybody’s hip* older cousin that doesn’t seem to show up at parties any more. Was he ever actually cool to begin with? *You can probably tell I was never hip, based on my usage of terms like, say, ‘hip’.*
My understanding of PCM products on the market today, (I’m thinking BioPCM or InfiniteR domestically, or any number of PCM products in Europe including drywall and apparently windows) are that a proprietary organic or inorganic material is sandwiched into a film or tile or ‘blanket’, etc. The material simply changes phases within some relatively small window of temperature, thereby absorbing energy/heat as a space warms and releasing energy/heat as a space cools.
This seems pretty straightforward to me. This also seems pretty great/useful/awesome/magical. The physics here don’t appear overly complicated. Simplicity appeals to me, as I suspect it does to many of you, because of course simple things often just work better.
All of that said, I would characterize the overall vibe toward PCMs here at GBA as lukewarm at best. Build your structure tight. Insulate the envelope. Control the water. Control the vapor. Use whatever cost effective solutions you can find to condition your space for health and comfort. ADD PHASE CHANGE MATERIALS TO REDUCE SWINGS IN TEMPERATURE AND INCREASE COMFORT???
What makes the last sentence wrong/silly/naive/uninformed? I’ve heard the use of PCMs requires large swings in interior temperature. But looking at the data sheets there sure seems to be a lot of stored energy released within a very small temperature range. I’ve heard the products are unavailable, but I’ve sourced multiple options to date domestically. I’ve heard the products are too expensive, but at $3.00/sf and 100+ BTU’s of energy storage/sf, a small, well insulated off grid cabin without the amps to run a mini-split in climate zone 5, (like say, Leavenworth, WA) sure seems to be a great use of the material. I’ve heard that PCMs are nothing more than expensive thermal mass, but this seems to gloss over thermal mass being a huge uncontrolled heat sink whereas PCMs only absorb/release heat within a very specific temperature window. I’ve heard that the packaging is suspect and apt to tear…which is probably true, but where is your sense of adventure, man!
Anyway, I’ve never built a home and I don’t even work in the building industry, so I feel like a virgin at the adult video industry awards. Who can set me straight? Assuming all the things we unambiguously agree on are done right, (air tightness, thermal envelope, vapor control, etc.) Should I take the $3k and invest in PV with a small mini-split? Or should I cast off the yolk of conservative building professionals and strike out into the wild blue yonder with nary a care in the world nor a concern for the pocketbook? Ah, the passions of youth!
I’m looking at you, God, I mean Martin Holladay. And you, Dana Dorsett, you angel of Northwestern wisdom.
~Humbled in Seattle
GBA Detail Library
A collection of one thousand construction details organized by climate and house part
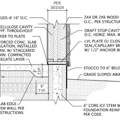
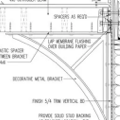
Replies
If your daily temperature fluctuates above and below comfortable room temperature, then some technology to buffer swings makes sense, you can increase comfort without spending part of the day heating and part of the day cooling. But if you spend all day heating or all day cooling, there's no advantage. You're better off just having an HVAC system that responds quickly to changes.
Most of the US, most of the time, you spend all day either in heating mode or cooling mode. Generally, when the daily average temperature is around room temperature, it's delightful out, and you can manage comfort just by opening windows.
Great point re: the daily temperature fluctuations above and below comfortable room temperature. The location in question, (Leavenworth, WA) is almost exclusively heating days October through May. June through September average lows range from 43 to 52 and average highs range from 78 to 87. I plan on using a tiny wood stove for space heating and it’s at least possible some extra buffer when releasing the BTUs in the winter will prove helpful. June to September in this climate poses an ideal fluctuation above and below comfortable room temperature which will hopefully make this whole concept workable...although that f*&%ing delta T may be ruining may day.
For PCMs to work, you have to accept a "significant" temperature swing between "warm" and "cool." How you define those terms matters quite a bit. For most Americans, a temperature difference on an hour-by-hour basis of more than a couple of degrees is uncomfortable. PCMs can't be dialed in that accurately. If you are comfortable with temperature swings of 10F+, then PCMs become more attractive. It's not just the amount of heat stored in the material, you still have to deliver that heat to the space. And with PCMs, the heat delivery is mostly via convection. Convection doesn't do much until you've got a few degrees of temperature difference. So there's a built in lag there. For something like a workshop, greenhouse, or other semi-conditioned space, this might not be too much of a problem. But for your living room it probably is. PCMs just don't have the regulation/control that most people demand for comfort.
Ugh, physics really is annoying sometimes. Why can’t anyone tell convection to deliver heat more effectively with a limited delta T??? Somebody should take this up with Martin Holloday…or god; whoever has control of this stuff. Anyway, your insight here is very helpful. I’m hopeful that with enough surface area/air movement/radiant heat transfer the PCMs will be able to remove/add heat to the space more efficiently than your experience and understanding suggests. I suspect temperature swings of 6F to 8F will be acceptable in this application. 10F+ will be tougher to swallow. I’ll need to dig into the spec sheets in more detail and run this issue up the flagpole with existing users of the technology. Thanks!
Phase change is used in some applications. For example, in places where electricity is a lot more expensive during the day than at night, people will make ice at night and then use it to cool during the day.
You're referring to so-called "ice chillers". You made ice during the night when electricity was cheaper during off-peak hours, then you thawed the ice out to cool your building during the day. This is a form of load leveling. In my area, these chillers are no longer cost effective because the difference between on- and off-peak rates has decreased in the past several decades. The utility wants to change this, but the regulators are resisting. The reason is that the utility would like to incentivise their larger commercial customers to do load balancing, which helps the utility with system planning. The regulators don't want to reduce the amount commercial rates subsidize residential rates (which they typically do), because it's the residential customers who vote for the regulators and their bosses. There is a conflict in interest here, unfortunately, which is a shame since I've always found ice chillers to be a cool way to economize on utility costs (no pun intended, BTW :-)
There are other ways to reduce cooling costs. Evaporative chillers are one. I had a customer once that used the "decorative pond" in front of their building to run their cooling system. Since they had a datacenter inside the building, they ran the cooling system all year. They mentioned to me how no one ever seemed to notice that they were the only building that ran their fountains ALL WINTER LONG...
The big issue with passive phase change materials is that the rate of energy transfer is tied to the thermal delta. This means the further you deviate from the mean temperature, the more the PCM acts, but it also means you have a "large" fluctuation in temperature that many people find objectionable. The best application I can think of for PCMs is where you have a semi-artificially increased temperature differential, such as tiles in a sunroom in a home. The sunroom lets in lots of sunlight, so the tiles are heated by this sunlight much more than they would by just ambient air. At night, the differential becomes much larger in the OTHER direction, so you get more useful heat output since they were essentially "charged up" to a higher temperature originally. This is lots better than just using these materials in something like an office hallway, for example, where there aren't any significant temperature swings.
Note that "solar greenhouses" use a similar concept, and some acutally do used PCMs. They regulate day/night temperatures this way, and since they can tolerate larger temperature swings, PCMs can be useful. I'm not aware of any normal occupancy buildings that use PCMs this way.
Bill
Sounds like your understanding of the issue here mirrors Peter Angle, namely that the delta T between ambient air temperature or surrounding materials and the PCM needs to be greater than is normally desired in a residential context. I will certainly need to find suitable solutions/mitigation of this issue if they exist. Thanks!
The electricity rates in WA are in fact quite low, (lots of low cost hydro, etc.) but this particular location is off grid and will stay off grid indefinitely, so I’m trying to limit my PV system to 24V if possible. Put another way, there are some additional cost savings if I can refrain from installing a mini-split or dedicated cooling equipment, etc.
If you can use 48v, you'll have greater efficiency over a 24v system.
I'm not sure PCM stuff is going to help you much here. I'd look into other passive techniques to limit heat gain, especially careful shading and extra insulation in places where it will do the most good. Reflective exterior surfaces can also help. The best balance of things to do depends on your specific situation.
Bill
I'm definitely going to do a deeper dive on the PV system, especially if I don't end up throwing in the towel on PCMs and instead go with an inverter and much larger storage, etc. to run a mini-split, (as I'm sure 95% of people would do in this situation). If I keep the runs super short and the wattage of any particular component pretty darn low, I may be able to get away with 24V power without the Amps getting too high. On the other hand, my wife will have something to say about my plans for our 'simple' cabin, and before you know it she may want a hair dryer and a refrigerator, in which case I'm screwed!
Re: shading, robust insulation, etc. we will absolutely work these angles. The house will essentially be a small airtight box with R45+ around and below and R80+ above, with moveable exterior thermal panels and appropriate shading of minimal fenestration, so if it were a little bigger it would likely meet the PHIUS standard. The PCMs are more of a technical curiosity that makes me want to try and get away without active cooling. I totally understand your skepticism and you may indeed be talking me out of pursuing this. Thanks for the additional input!
I usually drill a hole in the ice during heating season and fish out buckets of water to freeze in my oversized chest freezer. Warms the house nicely. I stash the ice in sawdust and tarps under the hemlocks for summer.
Come summer, I let the blocks melt in my sink. Cools things off a good bit.
I kind of wish there was a way to automate the process and make it more on-demand, but I can't think of anything real slick.
;}
In seriousness though, I find PCM's fascinating, but it's hard for me to intuit how a PCM window film could make that much of a difference (and at what cost?). I do sometimes wonder if PCM's might play a role in demand response solutions, but in that case I imagine it would be more about long-term/semi-long term storage rather than daily buffering, as I assume is the purpose of these film type products.
I fail to see how a PCM application in this regard is fundamentally different than thermal mass, save for the slim-downed nature of it. In both cases, heat is being stored. How would one create a PCM to NOT uptake or release energy save for a 'specific' temperature range? (I understand that it may undergo the phase transition and therefore have the most capacity for its size at a given temp range, but even before it undergoes phase transition it will be absorbing/releasing energy with the surrounding environment, no?)
edit: actually I guess I see how it would buffer in that temperature range most effectively, since you are adding to the environment a substance that maintains a flat line on the phase change graph at that temperature range.
>"I fail to see how a PCM application in this regard is fundamentally different than thermal mass, save for the slim-downed nature of it. In both cases, heat is being stored. How would one create a PCM to NOT uptake or release energy save for a 'specific temperature range?"
Physics says you can store a LOT more energy in a material when it changes state. This is why steam systems can move so much more heat around per unit of water than hot water systems can, for example. The same goes for refrigerants. That's the main advantage to phase change materials over more conventional sold thermal mass.
I don't know how you could create a controllable PCM, either, but that's really a problem for a physicist and I'm an engineer :-) Once a physicist comes up with a controllable PCM, I'm sure I could come up with a way to design it into a building to save money though. Engineers APPLY physics to practical problems. Physcists come up with the theory to give engineers the tools to solve those problems. We both usually get along because we agree this is cool stuff.
Bill
Yes, the ability to add heat without increasing temperature was apparent to me (which becomes very notable in the reduced size needed per unit mass) but I struggled to equate this with an increased buffering capacity during phase transition. I suppose it should be intuitive, but the explanation I think was touched upon when you mentioned the rate of transfer relating to delta T.
Because energy can be added to the system while the temperature of the PCM remains constant, a higher delta T between the PCM and the surrounding environment can be maintained for longer. Undergoing the phase transition doesn't itself increase the rate of energy transfer, but maintaining the delta T does. This leads me to believe that (as you also mention above Bill) a higher delta T (either due to sun radiation or artificially imposed) also increases the effectiveness.
It begins to feel like we ought to just use a refrigeration cycle at some point, but this has the advantage of being passive.
Given the above, coupled with the fact that it can be a much smaller package than other forms of thermal mass, I can see how it might have some niche applications someday.
As of now, I would question whether it is a necessary or costs effective element to design into a building when there's already sufficient tech to affordably regulate dwelling comfort. But if it proves to be both useful and affordable, then why not?
Install some of it Scottie and be on the bleeding edge!
Good points all around! In a misguided attempt to remove all cooling equipment I may just give it a shot! Likely to fail? Yes. Possible that we succeed? Possible!
Deleted
Your point re: short term vs long term applications of the technology is great insight. In this case the PCMs would be in tile or blanket form and likely live between the interior wall surface and the insulated studs, (also behind a 1.5” utility run). This is a little different than PCMs as a window film, which was news to me as well, and not a product that is available in the US to my knowledge. As part of this process I plan on crunching the numbers for replacing an oversized hot water tank connected to a solar thermal system (ala Thorsten Chlupp) with a PCM filled tank. Back of napkin numbers suggest a roughly 5x-6x reduction in tank size for the same thermal storage capacity. On the other hand water is basically free, (even if limited in off grid situations) and PCM bulk storage at this scale is more like $2/lb, so it can get pricey quickly. May be tough to justify and I still have lots of research to do on longevity of the material in this type of application.
It's not going to work as a passive system because you need a temperature delta in order to get heat to flow.
Here's a thought experiment to think about how it would work as an active system. Imagine you have a container of a substance that melts at room temperature and a heat pump. The heat pump is pumping heat in and out of the container as it heats and cools the space. If you're heating and the container gets colder than the outside temperature start pulling heat from outdoors. Similarly if you're cooling and the container gets hotter than the outdoor temperature, start dumping heat outdoors.
While you're using the container your heat pump is going to be more efficient than if you were using the outdoors, because the temperature delta is smaller. The question is, will you get enough use to make the expense and complexity of this system worthwhile? It depends on the size of the tank, your climate and your heating and cooling profile.
But to get an idea of how big the tank might have to be to be useful, think about this. The reason that air conditioner sizes are quoted in tons is that a one-ton air conditioner delivers the same amount of cooling per day as a ton of ice. Imagine that the substance you use has comparable heat of fusion to ice. If you had a one ton tank in your basement, it would give you a ton of cooling. That's not a lot.
Great points on complexity, cooling system size and reiterating the delta T issue. This does seem to be the crux of the physics issue, (although I’m sure there are other practical issues I’m too dense to think of). That said, this particular project will have a maximum heat load of ~2,900 BTUs/hr and max cooling load of ~1,200 BTUs/hr, (initial WUFI modeling) so the cooling requirement is roughly 1/10th of a ton. That’s still a lot of ice to store in your basement, but it starts to look a lot more like an amount of material you can hide behind every square inch of your walls/roof.
I know the University of Washington has two buildings using some form of this technology. The first basically takes a normal, fairly high functioning/well designed building and just adds some PCMs to the walls and roof of one portion of the building. The net outcome appears to be that the temperature swings are mitigated and the building is pre-cooled mechanically in the early morning without need for additional cooling on hot days, (some efficiency gains).
https://www.insolcorp.com/portfolio-items/university-of-washington-wa/
The second incorporates the PCM materials between each floor in a dedicated ventilation space that absorbs heat through the day and is subsequently vented at night. This apparently allows the building to fully function without any active cooling system. These buildings are in climate zone 4C, whereas the cabin I plan on building is in climate zone 5, but the same hot daytime and cool nighttime temperatures should be present during the cooling season.
https://phasechange.com/wp-content/uploads/2020/06/UofW_CaseStudy.pdf
Anyway, food for thought, and thanks again for all of the helpful feedback!
A ton of ice, if a cube, has an edge length of about 3' 3".
Not nothing, but not as bad as I first assumed.
Scottie,
Here are two items that might satisfy your PCM inquiry.
https://www.energy.gov/eere/buildings/thermal-energy-storage-webinar-series-novel-materials-thermal-energy-storage
Happy deciphering the one.
Hi Roger, thanks so much for sharing the video and slide show presentation from the NREL portion! This was a great overview of the research, practical applications and where the technology may head in the coming years. Based on the logarithmic graphs in the presentations of thermal conduction vs. energy density it appears salts are a better PCM material than paraffins for mediating space conditioning extremes and possibly for thermal (hot or cold) energy storage applications. I've discussed thermal energy storage options with a few PCM suppliers. Their level of sophistication to date appears to be, "Connect a heat source via a heat exchanger to the material and don't let the material get too hot too quickly." The presentation makes it obvious that different variations of composite conductive and PCM materials will change efficiency pretty dramatically in different use cases. I suspect this technology will advance quickly if the use cases are compelling enough to create demand. Cheers!
Key points so are:
1. It's only interesting when the daily temperature swings go above and below your target temperatures.
2. You need a delta T to get heat to flow in or out of your PCM.
On the second point, note that deploying the PCM in the drywall of the exterior walls can help even without much of a delta T between them in the inside space. Suppose the phase change is at 73 F. If it's 73 F in the room, there's no heat flow between the room and the PCM drywall. But if it's 40 F outside, and there's heat flowing out through the insulation in the wall, all the heat going through the wall is coming from the phase change, not the interior space. Then when it's 90 F outside, and heat in flowing inward through the insulation, the heat just goes to phase change in the drywall and not to heating the room. That works until the phase change is complete and then it stops helping.
But it's not clear that it's a better idea than increasing the thickness of the insulation in those walls.
Hi Charlie, thanks for the input! See inline...
"1. It's only interesting when the daily temperature swings go above and below your target temperatures."
I mostly agree here, although the more I'm thinking about this the more I think PCMs are a good match with a wood stove in a well insulated structure as long as the material's thermal conductivity is sufficient to absorb the excess heat efficiently.
Re: the delta T requirement and exterior wall PCM use, I suspect your analysis is correct re: PCM thermal energy flow acting similarly to additional insulation. The location of the PCMs within the thermal envelope will dramatically affect the amount of energy absorbed/released and the amount of time the PCM will effectively assist in insulating. I'm still trying to get detailed enough information on the thermal conductivity of different PCM products to model them effectively. If the PCMs can't absorb and release energy fast enough then they will do a poor job of adding to the insulative layer and will not effectively mitigate interior temperature swings. Delta T strikes again!
The following link is a fun thought experiment on the benefits of combining insulation and PCMs, (from a supplier, who conveniently leaves out the need to release the built up heat at night for the PCMs to maintain much value, but still pretty compelling [to me] when you do more in depth modeling):
https://vimeo.com/139916989
Heating with a wood stove is a special case. Wood stoves tend to suffer from two problems: the first is that you can't turn them down, there's a minimum size where wood burns well and it's pretty big. If you live in a well-insulated house it can be hard to keep a fire going and not overheat. Second, you have to feed them wood continuously.
These two factors mean that wood-burners spend a lot of time thinking about ways to absorb and store heat, so you can save it when you have too much until you need it. I've never heard of anyone using PCM's in that context, but I'm not an expert. You might try one of the forums devoted to woodburning like Hearth.com.
I'm not as optimistic as you are about this technology. A lot of people have put a lot of thought into the problem of storing heat. The science isn't new, it's been known for 250 years. There used to be a whole economy that revolved around ice, and as soon as electricity came around that dried up.
Indeed, my sense of it is that I’m 99% down the optimistic side of the spectrum here, and I’m not even so sure PCMs are going to work in this context. Duly noted re: the ice vs electric economy. Re: the wood stove, I’m envisioning using a combination of PCMs and DHW connection to the stove (which is a whole additional complicated ball of wax) to mitigate the overheating issue. There are fairly efficient small stoves in the US, 3kW - 5kW, that I’m hopeful will work in this context with 75-80% efficiency. The UK and Europe have even better wood stove options on the small end of the heat scale, but sourcing them in the US has been a tough uphill battle. This may all fall apart and I’ll suck it up, put in a big bank of PV and get a small mini-split...but I hope with enough research and effort I can find a different way. All of your feedback has been very helpful, so thank you for your honest assessments!
PCM's—liquid H2O to vapor—have been used 'forever' with woodstoves. People just let the vapor state run loose around the house :)
I suppose they would have to run a dehumidifier at night to extract that latent heat when wanted. Liquid to vapor doesn't seem the best phase change in this regard (though the latent heat of vaporization is significantly greater than latent heat of fusion for water).
Aren't most types of direct solar water heaters doing just this? Utilizing the increased delta T generated by the suns radiation and using heat of vaporization of an antifreeze?
Interior water vapor as heat storage, brilliant!
I'm no expert on solar thermal systems, but after doing a deep dive over the past month my understanding is that most systems are 'closed' systems where pressurized water or glycol moves between a collector and a storage unit and that vaporization of the glycol or water, (boiling) is a problem because the systems are pressurized. I suspect there are systems in place where the storage is not pressurized and can boil off as needed, which would allow phase change, although I'm not sure how much of this additional energy you'd actually capture. Cheers!
Back when people used to install thermal solar panels, "heat pipe" collectors were all the rage. The solar collector would be filled with a liquid that boiled around 85F. The vapor would rise into a heat exchanger, where it would condense and send its heat into water, and then drip back into the collector.
An issue with solar collectors is that their efficiency is directly proportional to temperature, they become less efficient at higher temperatures. However, the lower the operating temperature the more flow you have to have to get the same quantity of heat transferred. The heat pipe allowed greater heat transfers at low temperatures.
That video is interesting. Though it strikes me as similar rationale to using high SRI roofing, but with the possible downside of storing the energy rather than rejecting it (whether that's a downside or advantage would depend, but given the scenario conditions in the video, it seems like it'd probably be a downside, other than to help with possible condensation issue). At least for now, it probably also contains the downside of being more expensive than simply using a higher SRI roof. Am I off on this train of thought?
Also, it really only works the way he describes on a high heat gain surface (roof) during cooling season, right? It can't be used to reduce the delta from inside to out during winter in that way.
I think the concepts are similar in that reflectivity will directly affect the amount of energy/heat that ultimately travels through to the conditioned space. That said, the PCMs will in fact work to slow heat moving into the building in hot weather and heat moving out of the building in cold weather, so they have the advantage in that sense. On the other hand, reflectivity will work indefinitely, whereas the PCMs have a certain amount of BTU storage, (with most of these products around 100 BTUs/ft2) and once they have fully phase changed they simply act as additional thermal mass unless/until the energy is released. That last part is the big missing component in the video presentation, (from my perspective). Cheers!
Along those lines, you should compare the effect of PCM in slowing the flow of heat with just the same thickness (or even dollar amount!) of insulation. Just as reflectivity works continuously, so does insulation.
@DC Contrarian #34: A great point about the inherent advantage of additional insulation! This is further complicated by inputs in any modeling you do re: swings in exterior temperatures. If you make one set of assumptions, the same thickness of PCM is 60x more effective than insulation, (namely if you model sufficiently HOT days and sufficiently COOL nights). If you make a different set of assumptions, the same thickness of PCM is basically worthless compared to insulation, (namely if you model HOT days and HOT nights). With COOL days and COOL nights the PCMs still appear to add significant value when using a wood stove for heat. All of that said, the real take away here, (if you even buy into the concept of PCMs at all) is that the effectiveness is VERY situationally dependent. Also, (if you even buy into the concept of PCMs at all) the material still costs $2-$4/lb, so you definitely need to compare the benefits and costs. I'm probably still leaning toward incorporating the materials into our small cabin, if for no other reason than I'm curious and the costs will be rather manageable on a very small project, but I really appreciate all of the constructive feedback. Cheers!
Yes, a wood stove is potentially a good match for PCM. The stove runs much hotter than the desired room temperature, so you have an opportunity to have a PCM material with a phase change somewhere between the stove temperature and the room temperature, allowing sufficient delta-T both for charging and discharging. Compared to thermal mass, it can allow a couple of advantages. Too much thermal mass directly in the stove can inhibit getting up to a good temperature for clean combustion, and a thermal mass providing heat to a space will gradually provide less heat as cools off. The PCM can more rapidly heat up initially, before the PCM hits the the phase change temperature, and then can dispense heat at a consistent rate after the fire is out, until the PCM is depleted.
Many PCMs are flammable, which I'd want to avoid. A few studies I found use organic PCMs which seem problematic in that regard--I'd want to look at salts, and I think there are some in the right range (maybe 100 to 200 C)
https://www.researchgate.net/publication/333619990_Integrating_PCM-based_thermal_energy_storage_on_top_of_wood_stoves_concept_and_CFD_modelling
https://www.sciencedirect.com/science/article/pii/S1876610217358046
Exactly what I've been thinking! The two easiest PCM products to get here in the US, (that I'm aware of) are salt based, which is certainly a better solution than organics for high heat situations. Thanks for posting the PCM wood stove research, I haven't seen these previously. The specific recommendations for total volume of material and the need for air infiltration or conductive fins within the material for sufficient energy release is super helpful.
I'm thinking I will put the PCMs right in the firebox, cover them in lighter fluid, disconnect the exterior air intake to remove oxygen from the cabin, run an oversized bathroom fan to backdraft the flue, and subsequently remove the burning PCMs before dropping them on the wood floor to facilitate additional energy creation for storage by the PCMs in the roof structure. This way, if the CO doesn't kill me, the subsequent fire definitely will and my long suffering wife will get all of the life insurance money. We'll see what the researchers have to say about proper implementation here. Cheers!
"deploying PCM in the drywall of the exterior walls can help even without much of a delta T between them in the inside space. Suppose the phase change is at 73 F. If it's 73 F in the room, there's no heat flow between the room and the PCM drywall. But if it's 40 F outside, and there's heat flowing out through the insulation in the wall, all the heat going through the wall is coming from the phase change, not the interior space. "
-----
I'm having an exceedingly difficult time wrapping my head around this effect. I think I somewhat disagree, but I'm in the marsh.
The PCM (or any material) will always emit/conduct heat according to it's temperature with net flows according to relative surrounding temperatures. It seems the advantage of a PCM layer in the stack-up, as described in that vimeo link, is that it lowers the temperature differential across the assembly.
When the temperature point is suppressed by only a few degrees from what it would otherwise be, are we really getting much?
The PCM acts as a sort of balloon, expanding with latent heat, but not changing temperature. As a pressure analogy, this means the balloon doesn't increase in pressure as it fills with air (unlike an actual balloon). In this way, the PCM helps even out pressure spikes (temperature spikes). Continuing with that analogy, however, the balloon is not perfectly air tight. It still leaks air according to the material property of the balloon membrane (according to the phase change set-temp). In other words, it doesn't completely dam up the flow of air (heat) even while it is being filled.
Let's say normal drywall would hover around an average 71F for an interior set tempt of 72F.
As heat exits the building, it will pass into the PCM drywall. What happens now? What it doesn't do is raise the temp of the drywall. But does it get dammed up? While the energy is 'filling the balloon' with latent heat, the balloon is simultaneously leaking heat to the exterior, and it is doing so according to it's temperature (same laws of thermodynamics governing).
If the phase change is set to occur at 70F, then the PCM will buffer the temp down from 71F to 70F, and that is by how much the heat flow will be reduced.
To amplify the benefit, we would need to lower the design phase-change temp. If we lower it to, say 65 F, we've lowered the delta T a bit more significantly (though arguably still not of great value). But this means the PCM will more quickly balloon up to capacity (indoor design temp being 72) and if it does hit capacity, it will raise in temperature to the original 71 F, UNLESS the indoor temp drops frequently enough and with great enough magnitude to draw some of that stored latent heat back INSIDE (solidifying the PCM). But it only draws energy back in at a rate according to, once again, the delta T.
This certainly offers buffering capabilities, but it does not apparently reduce energy throughput in a steady state system by very much or at all.
It's possible I've completely bungled this, and if so please set me straight.
I think you need to look at the quantities of heat involved.
Let's replace your balloon metaphor with a reservoir holding water. Imagine that water flows into the reservoir, and you draw water out. If the amount of water flowing in averages out to the amount you take in the long run, the reservoir serves a useful purpose in evening out fluctuations in supply. However, if you take more out than is supplied, the reservoir will empty eventually. Similarly, if the inflow is more than you take the reservoir will overflow.
The kind of buffering that PCM provides is useful if the long-term net heat flow through the wall is zero or close to it. However, if the flow is mostly in one direction, it's like an overflowing reservoir -- what flows in is the same as what flows out.
When you start looking at heat-storing schemes, they typically have less than a day's worth of storage for a place that has real seasons. So basically, on the first day of winter you get the onset of heating season delayed by a few hours, and on the first day of spring you get a few hours of free heat.
But what about those days in the spring and fall when the outside temperature is both above and below room temperature on the same day? In theory, some sort of heat storage would make sense then. However, in reality, most people tend not to use any HVAC on days like that anyway. They just manage comfort by opening and closing windows.
@DC Contrarian #35: I think your take on the functionality of PCMs is accurate, and this also serves to frame the usefulness (or uselessness?) of the materials well. The point about just opening windows being sufficient in shoulder seasons is a pretty good one! In Climate Zone 5 with consistent diurnal heat swings during cooling season and the use of a wood stove during heating season, I think PCMs still have some compelling functionality if you buy into the concept and can mitigate the delta T issue. Thanks guys!
DC,
It sounds like you agree that it is mostly a matter of buffering. Ballast.
In addition to your notes about 'when' that buffering is useful, I would add that anytime a thermal interface sees a short term delta spike, there is an advantageous dampening effect. Very vaguely like using capacitance to suppress a voltage spike. Hence why the woodstove idea thus far seems the most promising, and why putting it near the exterior of a roof makes more sense than as drywall.
The energy from the spike, stored by the PCM, will then re-emit (in all directions) uniformly at a lower temperature, rather than going straight through the assembly as an unbuffered high-spike.
The water tank analogy is a really good analogy for typical thermal mass because as a water tank is filled with water, it's head pressure increases.
With a PCM, whether it's a tank or a balloon that we are analogizing to, we have to write in the caveat that pressure does not increase until it's full. I really can't think of a great analogy in that sense, but forgetting the analogy, all it means is that temperature drives remain flat throughout the 'filling' process (which is not the case with standard thermal mass).
I have no idea how much real advantage this offers over standard TM, but I suspect it's not very much (perhaps most when applied to sunny roofs compared to drywall, and that was really my point).
@Tyler Keniston #38. To summarize, look for higher delta T and take advantage of it! I like your thought process!
@Tyler Keniston #32: I think your example above is basically correct if energy hasn't previously been absorbed by the PCMs via a higher interior temperature. To put it another way, there is very little value to the PCMs unless they are actively working against energy transfer you don't want by releasing previously stored energy (keeping a well conditioned space from cooling faster to a colder outdoor temp) or absorbing excess energy, (keeping a well conditioned space from heating faster from a hotter outdoor temp). The basic take away, is that the materials may compound the positive effects of diurnal cool/hot cycles, magnify the effect of thermal heat gain in cold climates, mitigate and store excess heat from a wood stove and provide efficient mass thermal storage in lieu of water...if you buy into the concept at all. But I do think you're basically right, that unless the PCM has excess thermal energy stored it won't do much to mitigate heat losses with a 73F interior space and cold outdoor temp. Cheers!
Hi Scottie. I think we're basically on the same page. We all seem to want to frame this a little differently, but I think we're all circling the same ideas.
In my mind, whether the PCM has 'excess' thermal energy doesn't really dictate what happens to energy throughput at a given time. But certainly there is a time function involved here.
When plotted on a graph, if time vs temperature is a wave, any thermal mass will act as an oscillation dampener. By slicing off the peaks, the moments of maximum thermal deltas are indeed reduced. This seems to be essentially on par with what you're saying.
When taking a snapshot in time, in my mind it's easiest to simply describe the effect in terms of temperature difference. I think (but am not positive) that everyone is in agreement that the PCM only 'slows' energy throughout insofar as it reduces delta T.
All the time effects can be expressed in derivative form by this snapshot relationship.
@Tyler Keniston #41: I agree we're basically on the same page here, with a similar understanding of the physics and expressing concepts slightly differently. If the end point of any given thought experiment is that PCMs have the capacity to reduce temperature swings and absorb/release thermal energy without changing temperature, (sometimes in helpful ways) then I suspect the analysis is correct! Most importantly from my perspective, all of the input/links/feedback on this thread have helped me define whether these materials are even worth considering, and if so, which aspects/concepts are worthy of exploring further before deciding on a final design. If anyone has any interest, I'll share the results!
I am very interested in your results with PCMs assuming you went through and implemented it.