Thermal Mass and the Warming Climate

As many, I’ve been reading about the heat in most parts of the Northern Hemisphere right now. Feels like across North America, Europe and Asia, a vast portion of the planet is undergoing a heat wave at the moment.
As I’ve been reading about heat island effects in big cities, it got me to thinking about some of the passive heating techniques we talk about including building with high thermal mass materials to capture heat during the day and release them at night. Concrete floors or walls, straw bale or rammed earth walls, trombe wall systems, all operate on this premise that the day is warm and the night is cool, and the thermal mass will act like a giant capacitor, absorbing heat during the day and releasing it at night.
In theory this all makes sense, when you have warm days and cool nights. But as phenomena like heat domes become more common where stagnant hot air remains trapped over a region for days at a time and the nights don’t cool off sufficiently, can this technique really backfire and create more problems than it solves?
If the thermal mass can’t cool off for days on end, aren’t we just building our own little heat islands that will require more energy to actively cool them during extreme heat events?
Curious if others have thought about this and have perspectives.
GBA Detail Library
A collection of one thousand construction details organized by climate and house part
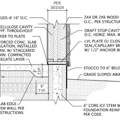
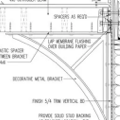
Replies
I think there's a distinction between useful and useless thermal mass, and a lot of thermal mass discussed is generally useless. I do think there's a place for useful thermal mass - more along the lines of phase change materials which may not cool down but will remain more constant. Likewise, controllable vs. uncontrollable thermal mass seems like an important distinction.
There used to be, in decades past, a sort of informal contest between passive solar, which made use of thermal mass, and so-called “super insulated” structures. The general consensus is that super insulated won the battle. That’s not to say that thermal mass can never be helpful, but you generally get more benefit from adding insulation than you do from adding thermal mass, and insulation doesn’t have the negative of slowing down the thermal response when you’re actively trying to change the temperature of a space.
Bill
Yes it works both ways. Thermal mass-based systems (sorry DC, that's been the term since the 70s) average out temperature swings. With most "high-mass" homes, at best you have a few hours of storage, so if days are 90° and nights are 70°, other inputs (gains and losses) aside, the interior will be around 80°. That's why I, and most modern high-performance practitioners, focus on air-sealing and insulation instead of thermal mass for temperature control.
"But as phenomena like heat domes become more common where stagnant hot air remains trapped over a region for days at a time and the nights don’t cool off sufficiently, can this technique really backfire and create more problems than it solves?"
While I 100% agree with the others that thermal mass isn't really helpful in your proposed situation, I'm not entirely sure it is specifically harmful. Could someone expand on the principle that would create increased energy usage in the above scenario?
Unless there is an efficiency quirk in the operation of the HVAC system I'm not considering, the only way I can see the thermal mass causing increased energy use is if more energy is entering the building by virtue of having the increased thermal mass.
Perhaps the thinking is that without thermal mass, people would be OK without AC at nights (when in the 70's) but with the thermal mass it would be too warm? If rather than keeping a rigid set-point with HVAC equipment, if people were willing to let their house get hot during the day when they are away, then open windows and get significant air changes at night to ride it without AC, then perhaps yes the thermal mass would be specifically bad at that point because it would slow the house from 'naturally' cooling via air change.
maine_tyler,
That makes sense to me, although Maxwell's original post throws a wide net as to what thermal mass we are discussing. Some like Rammed earth or Tromb walls can bypass insulation that otherwise would limit the interior heat gain. Maybe there is a distinction between how houses with lots of interior mass would cycle, and those designed to actively encourage heat gain?
The biggest issue is that you can't actively control thermal mass in a passive system (like a big concrete slab or similar "chunk of heavy stuff". This means that if you want your air conditioner to cool down your overly hot home, your A/C has to work to cool off and remove all the heat stored in that thermal mass before you'll start cooling down your home. The thermal mass works against you here. That doesn't necassarily mean you're using extra energy, since that is a more complex question. The same goes for heating too.
Where thermal mass could potentially increase energy consumption is if it's storing energy that would otherwise have been dissipated, such as energy coming into a home designed for passive solar. In this situation, that stored heat is extending your cooling cycle and using more energy compared to the same home with windows that would reflect more of that heat out so that the A/C never had to deal with it at all.
Thermal mass can only store energy, so it's a sort of thermal battery. It will delay drops and rises in temperature, slowing the transition from the high to the low or vice versa. Insulation slows the loss (or gain) of energy, so it acts to slow the change of temperature too, but it does it in a way that doesn't inhibit your ability to actively control the enviornment inside the structure.
Bill
Re-reading now, i realize my post was somewhat ambiguous and I should have clarified.
What I was specifically worried about was peak energy usage. In the midst of a heatwave, when the grid is straining under the combined demand of everyone running their AC units at peak power, would these homes built with a greater capacity to retain and store heat actually work against the occupant and demand more energy to be consumed to cool the home to an acceptable temperature.
I think I've read that as we work to electrify our homes, power grids have to be designed to meet peak demands, not average, and it's in these peak demands often that fossil fuel plants have to be fired up or ramped up on the grid to meet the need.
So even though you might build a house that behaves in a more energy efficient manner on average over the course of a year, might it actually create more problems than it solves due to peak usage in the midst of a heatwave.
Thermal mass can be modeled like buffer tank used in Hydronic system. You can store heat or cold in concrete or a large tank of water, but you need control how the heat (energy flows). Storing energy (or is absence), makes no sense unless you can control the flow or flux.
If hot stagnant air pools over a region, then you can could pump the heat to earth (geothermal), which sometimes is modeled as a heat sink for cooling. The downside to this approach is drilling down and costs for pumping. Air to water heat pumps can cool, but the rate is dependent on the outdoor air temperature.
Need to design a heat sink when you are designing the foundation.
Here's a good overview of thermal mass and how to calculate it: https://energyeducation.ca/encyclopedia/Thermal_mass.
One of the reasons I maintain that "thermal mass" is pseudoscience is that it has no units. That article says nothing about how to calculate "thermal mass."
It seems pretty clear that they are using the term Volumetric Heat Capacity (with the SI units MJ/m³K) as a way to describe the imprecise term Thermal Mass. It also seems clear that to find the volumetric heat capacity you multiply the specific heat capacity by the material density. Perhaps it's not explicit if you don't understand basic science and math, but you do, so you're just being obtuse.
As I've said before, I'm not a proponent of using thermal mass in my designs, but I'm also not pretending that it doesn't exist.
I find that when people bandy about "thermal mass," the meaning varies. Sometimes they're talking about weight, sometime density, sometimes heat capacity, sometimes specific heat. I don't think most people mean volumetric heat capacity, but hey, if nobody's right everybody's right.
Most people here don't seem to understand thermal mass, especially those who claim its pseudoscience (you). So why talk about what most people think, other than to keep everyone confused?
Please try reading the thermal mass article again and try to understand it.
Longtime readers know I get excised when people talk about "thermal mass." While I've heard that term used to describe a lot of different properties of matter, mostly people are talking about what is properly called "heat capacity."
Yes, it's important that a building have heat capacity. It's implicit in both the theory and practice of HVAC design that a building have heat capacity. The reason that Manual J targets the 99th percentile and first percentile rather than record highs and lows is that it is assumed that the building has enough heat capacity to handle brief excursions outside the rated capacity of the heating and cooling system without sacrificing occupant comfort. Similarly the way that HVAC systems are controlled -- with an on/off thermostat -- assumes that the building has some heat capacity, otherwise they would short-cycle.
Proponents of "thermal mass" believe that conventional construction lacks sufficient heat capacity, and that adding more is better. Even though for the most part this "thermal mass" is something that cannot be measured or calculated. And often the prescription for adding "thermal mass" is the liberal use of concrete, which is among the most environmentally destructive building materials.
Proponents often point to environments where the daily temperature swings above and below room temperature as good applications of "thermal mass." I'll point out that it is hardly an accomplishment to have a building with conventional construction maintain comfort in that kind of weather. I've never lived in a house that needed cooling during the day and heating at night. Where I live, in Washington, DC, it's normal for large buildings to have HVAC systems that have to be switched from heating to cooling, and typically that happens twice a year around May 1 and October 1.
Depending on construction, a four-bedroom house might weigh 100,000 pounds. The construction materials typically have a specific heat of about 0.50, which means that the house has a heat capacity of about 50,000 BTU per F degree. Compared to the heating and cooling loads a house typically sees that's substantial, and I'll argue that's sufficient.
This is a good explanation.
There are three problems with the idea that you can keep a building cool with thermal mass/thermal capacity. The first being that, as you say, you already have a bunch of thermal capacity and adding more might be pointless. The second, the origin of the question, is that you need the night temperatures to be low enough to provide substantial cooling. And the third is that in a humid climate, even if the night temperatures are adequately cool, the humidity might be near 100% and lack of humidity control can be problematic for many reasons.
There's a correlation between the second two issues: regions with high humidity don't drop to very low temperatures at night. Europe has always had substantially lower summer dew points than the eastern US, which is a big part of how they have been able to be comfortable without air conditioning, and why European building energy people sometimes think of night flushing as a good general purpose solution while in the US it's much more limited.
Nick and Charlie,
So would it be fair to say this argument is settled, and all that might be left to discuss is whether having some ability to store solar gains might make sense?
I'd like to think it's settled, but like perpetual motion it keeps popping up. Just this week we had a guy who posted who built an ICF building with effectively no insulation in the roof. He's gotten dried in and he's at the point where the trades are coming in, and he was shocked by the number the HVAC guy gave him -- he had been assured that since his ICF walls had so much "thermal mass" the building would practically heat and cool itself.
These misconceptions have real-world consequences.
Nick,
I meant settled among those of us in this discussion. Let's celebrate small victories when they occur!
Nick, your description of that other post is highly inaccurate. Please go re-read and edit your statements accordingly.
I'd like to offer a thought experiment as a way of thinking about this.
Imagine you have a styrofoam cooler and a block of concrete. You put the concrete in the cooler and set it outside. If you are so inclined, you could model the temperature of the block of concrete over time:
* In any time interval, the heat flowing into or out of the cooler is determined by the insulation value of the cooler, the temperature inside the cooler and the temperature outside. The insulation value of the cooler is determined by the r-value of the foam, the thickness, and the surface area.
* In any time interval, the change in temperature of the block is determined by the heat flow into or out of the cooler (calculated above) and the heat capacity of the block. The heat capacity of the block is determined by its mass and its specific heat.
What you would find is that the temperature fluctuations outside the cooler are greater than inside -- the fluctuations are dampened. If you increase the size of the block -- increasing the heat capacity -- or increase the thickness of the cooler --reducing the heat flow -- the dampening increases. You could also increase one and decrease the other and keep the dampening the same; for any level of dampening there are an infinite number of combinations of block size and cooler thickness that give the same amount of dampening.
If you increase the dampening enough you'll eliminate day/night fluctuations in block temperature, and going further you'll eventually find that the block settles down at the annual average temperature of the region [note 1]. Now, suppose further you're someplace like New England, where the average annual temperature might be 50F, and what you really want is your block to stay at a comfortable temperature like say 74F. You're going to need to hook up a heat source to keep your block at 74F when it's cold out, and a cooling source to keep it at 74F when it's hot out. [note 2]
Suppose you now want to model the behavior of the block. On days when it stays below 74F all day long, the amount of heat needed is determined entirely by the thickness of the cooler -- the insulation level. The size of the block doesn't matter. And increasing the thickness of the cooler always means you use less heat. Similarly, on days when the temperature stays above 74F all day long the amount of cooling needed depends entirely on the insulation level, and increasing the insulation level always results in less cooling being needed.
OK, but what about days when the temperature fluctuates both above and below 74F? If you tried to keep the block exactly at 74F you'd have to cool it every moment of the day when the outside temperature is above 74F, and heat it every moment when the temperature is below 74F. That might leave you thinking, "well, actually, I don't need to keep it exactly 74F. Plus or minus a few degrees is fine." So now you're targeting a range -- say, 70F to 78F -- and you only apply heating or cooling outside of that range.
You can minimize the amount of heating and cooling you have to provide if on any given day you provide just the net necessary for that day . On a day that requires net heating you need to supply that amount of heat no matter what, but if you don't also have to supply cooling that's all you need to supply. What you want is enough insulation and a big enough block to dampen out any fluctuations above the top of the comfort range. Once you've got that level of dampening, the heating required is determined entirely by the thickness of the cooler -- the insulation level -- and increasing insulation always results in less energy used. Cooling is exactly the same.
Recall also that for any level of dampening, the more insulation, the smaller the concrete block needs to be.
So to summarize:
* In the heating season the thicker the insulation, the less energy you use. The size of the block doesn't matter.
* In the cooling season the thicker the insulation, the less energy you use. The size of the block doesn't matter.
* In the "shoulder" season, the thicker the insulation, the less energy you use. The block and the thickness of the cooler have to be chosen so that sufficient dampening is provided; the thicker the cooler the smaller the block needs to be.
I hope you see that in these hypotheticals, the cooler represents insulation and the concrete block represents heat capacity (or "thermal mass" if you must). I hope you also see that as houses get better and better insulated the heat capacity becomes less and less of an issue.
Notes:
1. This is essentially what a cave is like.
2. I'm assuming it's not really practical to dampen fluctuations on a longer scale than a day.
How about a real life experiment? YOU TOLD ME to do an experiment. Build a house with thermal mass, then build a second identical house, except without thermal mass. Compare the differences.
I pointed out to you that I already did that sort of experiment. I built a two story house, with both floors, same dimensions and insulation values. One had a tiled slab floor thermal mass, and the other had a carpet over ply subfloor low thermal mass. The results showed that the thermal mass dampened internal heat fluctuations by about one half vs. the low thermal mass condition. That is, typical temps ran 68 to 78 year round with thermal mass and direct gain solar heating only, versus the low mass of 65 to 83 during winter. Oh, and a third of the time in winter, we had to use backup heating overnight in bedrooms in the low mass level. So the results would have been worse for the low mass condition, without that extra help. The lower temps would have been even lower.
So for the REAL experiment that YOU PROPOSED, it shows that thermal mass has an effect on dampening temperature variation substantially. This is commonsense general knowledge anyway. And its been demonstrated over and over in attempts to build passive solar homes that store heat from solar gains that can be spread overnight, and those without thermal mass that reduce auxiliary space heating during the day, but need auxiliary heating to maintain comfortable temperatures by the early morning hours.
By the way, during an unusually long three day long winter heavily overcast period, the effect of the thermal mass showed 3F lower interior temperature minimums each successive day. (In five years, I only used auxiliary heating on the thermal mass level once, to see its effects, and none used during this period.) The effect doesn't "last one day." In the most stunning period, we have five days in a row of outdoor temps from minus 15F to minus low single digits with no auxiliary heating used on the thermal mass level. Interior temps dropped to 65F each morning, but bounced back into the low 70's F from the solar gains. Rather impressive, don't you think? (Heating was used upstairs in bedrooms with minimal thermal mass.)
Of course that was in sunny, high altitude Colorado, nearly the ideal climate for passive solar. But it CLEARLY DEMONSTRATES the usefulness of interior 4" concrete slab thermal mass. Which also functions as a floor.
Don't get me wrong. Passive solar isn't appropriate for all climates or building lots. Cold, sunny or mostly sunny winters are needed. For details, see:
https://www.greenbuildingadvisor.com/article/a-quantitative-look-at-solar-heat-gain
Warm climates, overcast winter climates lack the need or the solar gain for passive solar to work. Climates far north in Alaska, Canada and Scandinavia don't get sufficient winter sunlight to heat a building without absurd amounts of insulation. Lots that don't have unshaded solar access midday in winter (when the sun is lower on the southern horizon midday) won't get direct sun through south facing glazing to capitalize on solar heat gains. And passive solar buildings need to be reasonably airtight and superinsulated to have a low winter heating load. Sunlight isn't THAT powerful in cold northern climates.
High mass walls have been used in the Southwest USA successfully too. But I don't have expertise in that type of construction and haven't lived there.
And airtight, well-insulated buildings work well without reliance on wintertime solar heat gains. That's something that can be engineered to work in just about any climate that requires wintertime heating or summertime AC.
And what do you do? Ignore those results. Just keep claiming that thermal mass is pseudoscience, a perpetual motion type idea that doesn't work, that people who know it works just "believe in magic." And you confuse thermal mass with weight, though there's a correlation there. But steel is heavy and concrete is heavy, both are low R-value, but only one of them works well as thermal mass. You claim there is no measurement for thermal mass, even when the comment JUST PRIOIR TO YOUR CLAIM showed measurement units.
I've asked you over and over about why you persist in claiming thermal mass is pseudoscience, given the results of YOUR EXPERIMENT that showed its usefulness. Common knowledge anyway. Real scientists propose experiments when there's disagreement about theory. We did the experiment, and the results were unambiguous. Lots of others have done the same thing. Your pseudoscience theory is disproven. As was obvious anyway.
And you just keep demanding that everyone is wrong and you are correct.
We don't need to discuss air sealing details with people who insist buildings need to breathe.
We don't need to discuss optimum levels of insulation with people who insist 2x4 walls with fiberglass are good enough for northern Michigan.
We don't need to discuss thermal mass with people who insist its just pseudoscience akin to perpetual motion machines.
That's a waste of everyone's time.
Two stories on the same house is not at all like two side-by-side houses.
In particular, one floor has conditioned space above, the other has a roof. It's not at all unusual or remarkable in conventional construction for the upper and lower floors of a building to have different performance.
So why does the upper story get hotter if you think its less insulated? Why would it get HOTTER??? Why does the upper story get colder if you think...whatever. Heat FLUCTUATIONS is the dependent variable in this experiment. One is double (not 5%, not 10%, its 100% greater fluctuations) vs. the other with the same weather, same walls, so many similarities except one big difference. Thermal mass. Not only one case study here. So many others have noted this effect. Are all these people WHO DID THIS EXPERIMENT all wrong, but you are right?? I didn't invent this passive solar design, I just did a good job engineering and building it. Lots of books told me how. A course on ASHRAE Handbook of Fundamentals and passive solar design educated me on calculations. They are ALL wrong?
Man up. OR admit you are the one who preaches pseudoscience.
Naturalistic experiments are valid. Just like studies that noted when the 65 mph national speed limit was instated, highway deaths went down significantly. Lots of difference before and after besides the speed limit. But it is clear evidence that lowering the speed limit results in fewer traffic fatalities.
Nick,
As I said in my post above, I think your hypothetical does a good job of describing how thermal mass (sorry) isn't effective when considering it's relationship to an insulated building envelope. But it doesn't deal with how it interacts with intentional solar gain. Whether that's worth designing in in most climates is another question, but it does seem to require a different conceptual analysis than the one you gave.
When I finished post #17, I thought, "I bet someone's going to ask about solar gain." Thank you for obliging.
In this simple block-in-a-box model you can think of solar gain as additional heat that gets injected into the system. The additional heat means that some days that were heating-only now become shoulder days. Since all the heat comes during the hot part of the day you don't get any shoulder days becoming cooling-only days. So you have more shoulder days.
Solar gain doesn't change the analysis of heating-only or cooling-only days.
There are two important changes to shoulder days with solar gain:
The shoulder days become more extreme. This means you need more dampening. Since the solar gain is coming during the hot part of the day, the difference between the heat gain in the hot part of the day and heat loss in the cold part of the day becomes greater. So a level of dampening that was adequate in a building without solar gain may now be inadequate.
Insulation does not dampen solar heat gain. Only heat capacity does. This is a big one. Insulation slows the heat gain when it's hotter outside than in, but it does nothing to keep the sun from shining in. The dampening of the heat from solar gain can only happen from heat capacity. When do you need to dampen solar heat gain? On heating-dominant shoulder days -- days when you run the heat at night but not during the day. On cooling-dominant shoulder days -- when you run the AC during the day but not at night [note 1] -- the night-time dampening needed is the same as without solar gain, there's no solar gain happening at night, presumably the dampening is adequate. But on heating-dominant shoulder days you want to have enough heat capacity to your house so that you don't overheat when the sun warms things up -- although only so you achieve the goal of not running heating and cooling in the same day.
But there's a caveat: with solar gain, a heating-dominant shoulder day becomes a cooling-dominant day if you add insulation!
So there's not really any cut-and-dried answers. While I've tried to be descriptive and not prescriptive -- and none of this is quantitative -- I hope I've laid out the roadmap of the analysis you'd have to do in the design of a specific building.
Notes:
1. On shoulder days you often don't have to run the AC, just opening a window provides sufficient cooling.
The thermal mass overviews are charming, but to circle back to the original question and reiterate a point that's been circled around a bit:
"If the thermal mass can’t cool off for days on end, aren’t we just building our own little heat islands that will require more energy to actively cool them during extreme heat events?"
Thermal mass (heat capacity) in a building that simply buffers temperature swings should not be conflated with heat island phenomena or with solar gain.
'Thermal mass designs' that increase solar gain would certainly be problematic in extreme heat scenarios. Thermal mass that simply acts as an internal heat buffer—and when cooling is performed actively as stated—does not have a fundamental detrimental effect on the energy budget.
Practically this might mean that one so inclined to build high mass walls (low thermal diffusivity) should not shy away from doing so out of fear of creating a sort of micro heat island effect.
I imagine that the question stems partly from 'heat island causes' google results often listing the thermal mass of city structures as a cause. Unfortunately, most of these top google results don't properly address that issue but simply place it as an item in a short bullet list.
https://www.epa.gov/heatislands/learn-about-heat-islands#causes
^This page does a decent job explaining how the 'thermal mass' factor affects heat islands, stating one of the causes as follows:
"Urban Material Properties. Conventional human-made materials used in urban environments such as pavements or roofing tend to reflect less solar energy, and absorb and emit more of the sun’s heat compared to trees, vegetation, and other natural surfaces. Often, heat islands build throughout the day and become more pronounced after sunset due to the slow release of heat from urban materials."
In other words, these materials are actually absorbing more heat, not just time shifting. But time shifting is also provided by the increased heat capacity causing the effect to be pronounced in the evening/night.
Thermal mass in and of itself doesn't add to (or reduce) energy use. Thermal mass acts like a battery -- you only get out of it what you put into it. In this way, thermal mass SLOWS temperature changes, and DELAYS the onset of the maximum high or low temperature.
Where things get interesting is when that thermal mass is combined with other things (like passive solar concepts letting the sun heat up the thermal mass), because now you have a potentially external source of energy coming in that could potentially change things on your energy consumption side.
I don't think we'd have a 'heat island' effect, but you could potentially make your A/C seem less effective by making it take longer to bring down the indoor temperature. Most of the discussion here is centered on thermal mass used INSIDE of structures. The urban heat island is with OUTDOOR thermal mass, such as pavement or a big parking garage. There has been some interest in this, since urbanization of some areas of historical temperature measurement has resulted in the thermal mass keeping the area around the sensor longer into the night, which results in the average temperature of the area reading higher. There has been some concern that this effect results in an overstatement of warming trends in some areas.
Bill
I have to admit to being slightly confused Bill when you respond with nearly identical statements as the post you are replying under. Are you just corroborating the sentiment, or are you trying to refute something? Either way I appreciate hearing from you and if it's the former, great! If the latter, I just can't tell what you are trying to refute or clarify.
Maxwell,
To answer your original question "If the thermal mass can’t cool off for days on end, aren’t we just building our own little heat islands that will require more energy to actively cool them during extreme heat events?"
Everyone should understand that “thermal mass can’t cool off for days” isn’t what thermal mass does in general. I don’t think that’s what Maxwell meant, but note: Thermal mass will absorb and release heat by definition. So for city streets or concrete buildings, which have higher thermal mass, thermal mass will absorb heat during the hotter periods (removing heat from the surrounding air), and release heat during the cooler periods (giving off heat to the surrounding air). Thermal mass reduces the highest temps of surrounding air, and increases the temps of the coolest periods. Thermal mass can accumulate more heat during multi-day periods, or seasonally during summer, and give off heat during cooler hours, cooler days, and the cooler or colder months. It will heat up and cool off significantly over the seasons, and between the daily outdoor highs and lows. Low thermal mass materials will heat up and cool off faster than high thermal mass materials. Thermal mass temperature will increase and decrease, but that heat is transferred from the surrounding air.
Thermal mass does not add heat or cooling. It doesn’t make cities warmer by itself. Thermal mass dampens heat fluctuations. That means high temps aren’t as high, and low temps aren’t as low. Maxwell only mentions the warmer nights during the hottest weather periods, since typically nights are cooler than daytimes if the weather pattern isn’t changing for days. But he didn’t mention the cooler daytime temperatures as thermal mass absorbs heat higher than its temperature on the hottest days. Thermal mass works both ways. Daily high temps wouldn’t be quite as hot, and daily low temps wouldn’t be quite as low, during an average heat dome weather event. Or any other weather event. Typically, the daily low temperature is sometime before dawn, and the daily high is in the afternoon, but it depends upon the weather pattern. A significant amount of thermal mass would reduce the variance of these highs and lows if the surrounding air is fairly stagnant.
So thermal mass might extend the duration of heat waves, but it also reduces the effect of the initial heat wave as cooler thermal mass absorbs that heat. The more the temperature extremes between the surrounding air and significant amounts of thermal mass, the greater the effect of dampening.
To reduce heat islands in cities, common solutions propose shading or more reflective material to avoid heating the area. Green roofs and cool roofs. Trees and vegetation cool the air through transpiration.
To be clear, thermal mass itself is not the cause of hotter cities. You can blame global warming, fossil fuel overuse, too many vehicles emitting heat, materials that absorb too much solar radiation, or other factors. But thermal mass doesn’t add heat or reduce heat. And I haven’t noticed any proposed solutions to build low thermal mass roads and lower mass buildings to reduce heat dome effects. They would backfire when heat domes drive up temperatures in cities more quickly to even hotter temperatures, although temperatures would also drop more quickly to even lower temperatures.
More trees, vegetation, less energy usage in the city, maybe using more public transportation and fewer cars with only a single person driving in traffic backups, distributing arrival times for workplaces, more remote work and less commuting, moving heat generating industry and processes out of the city, more reflective surfaces facing the sky, surfaces that radiate more heat out towards space. I’m sure all of you have heard of good ideas that could generate less heat in metro areas, if that is your concern.
For more, see:
https://www.epa.gov/green-infrastructure/reduce-urban-heat-island-effect
This is really well stated. Thank you.
I guess my concern really arose from this idea that there are maximum temperatures that the human body can handle. If the average air temperature around us approaches 100F and stays there for days on end, that can kill people (and has in many cases).
So while I appreciate the idea that a high thermal mass building would keep the occupant cooler than the surrounding temperature at the start of the heat event, by day 2, day 3, day 4, etc. of consistently high temperatures, would that effect not disappear and now the occupant is in a space that is actively fighting against him/her and his/her efforts to cool it?
And in a world where our energy grids have to be designed to handle peak loads moreso than to address average loads, are we creating more energy demand from these homes during those brief (but becoming longer and more frequent) periods of extreme heat that may cancel out or reverse any energy saving / carbon saving benefit they may provide for the remainder of the year?
(And I specifically mention carbon saving benefits because my understanding is that during peak energy load scenarios is when coal and NG power plants tend to be ramped up to full capacity and produce the greatest emissions even though the grid may be relatively clean and mostly renewable-powered the rest of the year.)
Maxwell,
I may not be fully understanding your line of thinking here, but it seems you are still missing part of the picture if you think thermal mass is causing more energy to be expended during heat spells.
"would that effect not disappear and now the occupant is in a space that is actively fighting against him/her and his/her efforts to cool it?"
You seem to think that thermal mass only takes on heat and doesn't simultaneously release it. Or you are still confusing the absorption of heat energy into an object with thermal capacity.
"And in a world where our energy grids have to be designed to handle peak loads moreso than to address average loads, are we creating more energy demand from these homes during those brief (but becoming longer and more frequent) periods of extreme heat..."
You are right that peak load matters a lot when it comes to designing the grid. But thermal storage doesn't add to peak load. If anything, it reduces it.
The only reason I can think that the sole property of heat capacity in a city would add to the total energy use is due to human behavior: if the heat capacity shifts the heat into a time when people want their dwellings to be cooler and so AC's are ultimately run at greater capacity on average.
>"So for city streets or concrete buildings, which have higher thermal mass, thermal mass will absorb heat during the hotter periods (removing heat from the surrounding air)"
>"But he didn’t mention the cooler daytime temperatures as thermal mass absorbs heat higher than its temperature on the hottest days. Thermal mass works both ways. Daily high temps wouldn’t be quite as hot, and daily low temps wouldn’t be quite as low, during an average heat dome weather event."
I think you understand this full well Robert, but I think its worth clarifying that its only the 'property' of thermal mass that is reducing peak temps, not the 'objects' themselves. In other words, the fact that all that thermal mass in a city is exposed to solar radiation and is absorbing more heat than would otherwise be the case leads to a net warming (even at the peaks), not cooling. Though one may call that concrete and asphalt 'thermal mass,' it is causing higher peak temps if it is absorbing sufficient additional energy. The 'property' of heat capacity does indeed mean that the absorbed heat is stored in the materials rather than released more immediately as sensible heat.
Which gets me thinking, what's an example of a material with a low albedo and also a low heat capacity. I suppose in theory such a material would contribute most immediately to higher sensible temps?
>Which gets me thinking, what's an example of a material with a low albedo and also a low heat capacity. I suppose in theory such a material would contribute most immediately to higher sensible temps?
Temps are always sensible. So just higher temps.
Think about what happens when sun hits a sheet of asphalt lying on the ground. Some of the sunlight is reflected, a lot is absorbed. The asphalt heats up. It transmits heat to the air above, and to the ground below. The heat from the sun is arriving on the top surface of the asphalt. The insulating value of the asphalt and the ground below limit how much heat flows down, and the heat capacity of the asphalt and the ground below determine how much temperature rise that heat flow causes.
If you wanted all of that heat going into the air the thing to do is to insulate between the collector and the ground -- or at an extreme, lift the collector off of the ground. Off of the ground, all of the heat that the collector collects is going either into the air or the collector. If you minimize the heat capacity of the collector you maximize the share that goes into the air. So the question really becomes what is the lowest heat capacity material that can be built into a free-standing structure? I'd guess aluminum or carbon fiber.
Note that albedo is entirely a surface phenomenon, so the collector could then be coated with a thin layer of low-albedo material.
I'm not sure what the usefulness of this would be.
I haven't read all of the above (TL:DR) so I risk the wrath of some posters, but...
Regardless of all the issues raised above, a well controlled thermal mass could be very useful in this era of peak-shaving and load shedding, that is Demand Side Energy Management. If you can effectively cool or heat a thermal mass when energy is cheaper you may be able to save significant money. You may also be able to help your favorite utility manage its load peaks, avoid brown-outs and help those who can't afford a sophisticated energy management system.
Energy usage, per se, is no longer the only consideration.
Any thoughts on how to implement such a system cost effectively?
Please read my post #17 for an explanation as to why that may not save as much as you think.
Nick,
As I understood #17, over the long term, the temp of the thermal mass will approach the average temp of the local environment and if you want a different temp, you have to remove or add some energy. Not only that, every time the temp of the thermal mass is different from the local temp, you're losing energy to the environment. Agreed. TANSTAAFL
My point is that if the price of that energy fluctuates somewhat predictably, you're aware of the fluctuations & their pattern and have control over the timing of your purchases, you can buy low and sell high, on average. There Ain't No Such Thing As A Free Lunch, but there are less expensive lunches. And unlike BLTs, BTUs are fungible.
bobbomax,
Count me among the posters who don't engage with people who write TD:DR
Malcolm, I expected some wrath. If you're able to parse 10 screens of verbiage, more power to you. I usually quote Feynman, "If you can't explain it to an 8 year old, you probably don't understand it."
The property of matter that determines how much energy it absorbs from solar radiation is called "albedo." It's expressed as a unitless measure between zero and one, the ratio of energy reflected to energy received. So a body that absorbs all of the energy falling on it has an albedo of zero, and a body that reflects it all has an albedo of one.
The reason that cities form heat islands is that they have lower albedos that what would otherwise be there. Just about every place where humans live the landscape is otherwise covered in vegetation. Photosynthesis happens best at temperatures between 70F and 90F, and the leaves of plants are highly evolved to reflect enough energy to keep their temperature in that range during the times in their environment when photosynthesis would be most effective. It's the original passive solar.
The things that we build cities out of tend to have lower albedos than plants, so they absorb more heat. Interestingly, it seems that Old World cities have higher albedos in general than new world cities.
I find the notion that a city has significantly more heat capacity than what underlays it questionable.
What's under a city? Dirt, stone, sand. Those materials all have specific heats in the same range as common building materials. If the city weren't there those things would be at the surface.
It's the heat absorption, not the heat retention.
Soil acts to insulate the bedrock beneath, from both heat loss in the winter and from heat gain in the summer. This is very apparent if you do any underground work in the winter and you can see how far down the frost goes. Snow also acts to insulate the ground, in this case reducing the frost depth. This stuff comes into play determining when the underground season ends in the utility world, since the frost depth eventually gets deep enough that it is no longer practical to do underground work except in emergency situations where we have to dig our holes with hot water and steam ($$$ !!!).
Paving with concrete I would assume (no numbers here) will hold heat well, but will be somewhat more reflective to sunlight. Asphalt I would expect would warm up more from sunlight, and probably hold a similar amount of energy as stored heat (again, no numbers here). Obviously what is built on any given plot of land has an ability to affect how much solar radiation is held onto in the form of stored heat, both based on reflectivity and on thermal capacity (specific heat) of the material.
I don't have a lot of real data here, just some general "physics says it outta work kinda like this". I do know you can make a measureable difference by painting things lighter colors as I have painted roofs of some large telecom facilities white to reflect more sunlight to keep indoor temperatures down, and it does make a measureable difference. There are even specialized coatings for this purpose, although I've never used anymore more fancy than a basic white primer. You can also measure the deterioration of the coating's ability to reflect solar energy over time as it degrades, gets less shiny, and gradually darkens as it ages and gets dirty.
Bill
Nick,
I don't think that "a city has significantly more heat capacity than what underlays it" is a claim I have seen made. As you stated yourself, where humans haven't built cityscapes, the land is typically covered in vegetation, so the dirt, stone, etc. really isn't quite at the surface. With vegetation there is more reflectance and also transpiration.
And so (in agreement with you) the real issue is energy absorption. I mean, considering the oceans and their vast thermal capacity, obviously the 'heat island' issue isn't really heat capacity. I think some 'what causes heat island' resources make erroneous claims about heat capacity, but others are just stating that it plays a role in shifting warmer temps into the night because the materials that have absorbed the additional heat have some heat capacity (and more than most vegetation I imagine).
Side note, I'm sure some here are already familiar with this, but here is a cool calculator that let's you calc how much energy various materials can store (calculator at bottom).
https://www.engineeringtoolbox.com/sensible-heat-storage-d_1217.html
Storing what I would consider significant amounts of energy in medium to large water vessels is certainly possible, physics wise (rivaling Tesla power walls). I think the bigger challenge is cheaply and effectively extracting or rejecting heat to/from that vessel when needed.
maine_tyler,
I know there are a lot of variables, but do you have a sense of what volume of water would be needed to make a useful contribution to a house's energy needs?
My only experience in anything remotely similar has been using cisterns fed by rooftop collection as the primary water source for houses here on Vancouver island. The problem we bump up against is the cost and logistics of building a suitably large storage tank.
The idea does have a long pedigree. I remember reading William Shurcliff's Super S0lar Houses in the early '80s, but my understanding is he later abandoned the solar storage in favour of better building envelopes.
Malcolm,
I certainly have no real world experience designing or building any such system (or more importantly, determining whether they SHOULD be designed and built). So the short answer is, I don't know, but I trust that we have arrived at favoring building better envelopes for good reason. Though part of that reason may have to do with the price of energy, as DC says, and other economic/industrial factors— making it not entirely technical in nature. (To be clear, I mean the additional use of storage. I don't think it would ever make sense to use storage in lieu of better envelopes.)
I imagine the answer depends a lot on what we consider a useful amount of energy to store. Are Tesla power walls a useful amount (around 13.5 kWh)? I think the real value to any sort of storage scheme in that ballpark , if there is any, is load shaving/shifting. Not so much long term energy storage.
When it comes to thermal storage, the other major factor is the delta T. Much 'ink' has been spilt about that here before as you know. Using the calculator I linked, one can plug in different delta T's and see how the total storage capacity of water changes. A big problem with any passive system is the difficultly in getting the larger delta T and controlling the timing/magnitude of the flow. An active system that can pump heat to and from the storage tank would allow a much higher delta T and more control (value). The water tank would still be quite large compared to a Tesla powerwall of course. And at that point, are we much better off than a ground source heat pump which uses the ground as the 'storage tank.' Many factors for sure.
But just for fun: to get to 13.5 kWh of storage capacity with water, if we allow ourselves to use a 40 degree temperature swing (not sure if that is reasonable to assume) then we would need around 80 gallons. Using 20 degree swing, double.
The problem with energy storage is energy is just too cheap to make it worthwhile.
Here's hypothetical: imagine you could make free ice in the winter and use it for cooling all summer. The alternative is to use an AC with a SEER of 20 and electricity that's $.20 per kWh.
A ton of ice delivers 288,000 BTU when it melts. It's easy to calculate the electricity needed for the same out of cooling, because the units of SEER are BTU/Wh, so just divide by SEER -- 20 -- to get Wh. That gives 14,400 Watt-hrs, or 14.4 kWh. At twenty cents per kWh, that's $2.88 worth of electricity. Let's say you pay $100 a month for cooling for four months a year, or $400 per year. You'd need 138 tons to get through the cooling system.
There's just no way you could come up with a way to store 138 tons of ice for half a year to save $400 and have it pencil out economically.
That's a really good point about the price. I will point out that one needn't store that many tons of ice if the goal is to shift the energy usage and not use 'free' energy (like ice from a pond). If instead we are allowed to pump energy into/out of a single storage system. This of course requires energy inputs, so it still may not 'pencil out' (more likely perhaps if time of use billing became a significant thing-- though grid level storage may very well be the sensible way forward). It at least makes it seem more practical in todays society where storing 138 tons of ice gets you side eye.
Here's a good article from NASA on why heat islands form:
https://mynasadata.larc.nasa.gov/basic-page/urban-heat-islands-background-information
From the article:
"The hottest places on Earth have a few traits in common. They are full of rock and stone, they do not have a lot of water, plants, or trees, and they are full of dark colors.
"Cities are full of these rocky surfaces — asphalt, brick, and concrete — that absorb heat by day and release it at night. These materials are used to make the sidewalks, parking lots, roads, and basketball courts of urban areas. Urban heat islands form because humans replace cooler surfaces with rocky surfaces.
"These hard and dark-colored surfaces contribute to the urban heat island effect in two ways. First, these surfaces have a low albedo, which increases the amount of energy from solar radiation they absorb. Second, these surfaces do not contain much water to evaporate, meaning that less of the absorbed energy evaporates water, and more goes into warming the surface and releasing energy by conduction, convection, or radiation. The combination of these factors means that cities and other highly developed areas are hotter than the plant-covered countryside."